The chemical bonding in 2-dimensional (2D) MXene material Ti3C2Tx and its precursor Ti3AlC2 was studied at beamline Balder. The relatively newly discovered MXene materials have potential applications from batteries to electronics. A team from Linköping performed the first experiment at Balder in 2019, and the results are now published in Physical Review Research.
2-dimensional (2D) materials for energy
Materials in the form of a sheet, one or a few atomic layers thick, are called 2D materials. The most well-known 2D-material is the single layer of carbon-atoms called graphene. However, there are many other both single atom and compound varieties. A class of 2D-materials that attract a lot of scientific interest are MXenes. The M stands for an early transition metal, the X is carbon or nitrogen, and the T is the surface termination, the kind of atoms sitting as the outermost layer of the sheet.
“There is strong demand for improved anode materials in, for example, lithium-ion batteries with higher capacity, better compatibility with electrolytes, and less sensitivity toward cycling losses compared with the graphite anodes used today,” says Martin Magnuson, associate professor at Linköping University. “The future of MXene-based batteries is particularly exciting.”
From MAX-phase to MXene
The raw materials for MXenes are called MAX-phases, which are a number of Mn+1Xn layers bound together by an A-element. The A-element is from IIIA or IVA group in the Periodic Table and could, for example, as in this case be aluminium. By exchanging the A-element for the Tx-element, the MAX-phase layers will separate and form 2D MXene sheets.
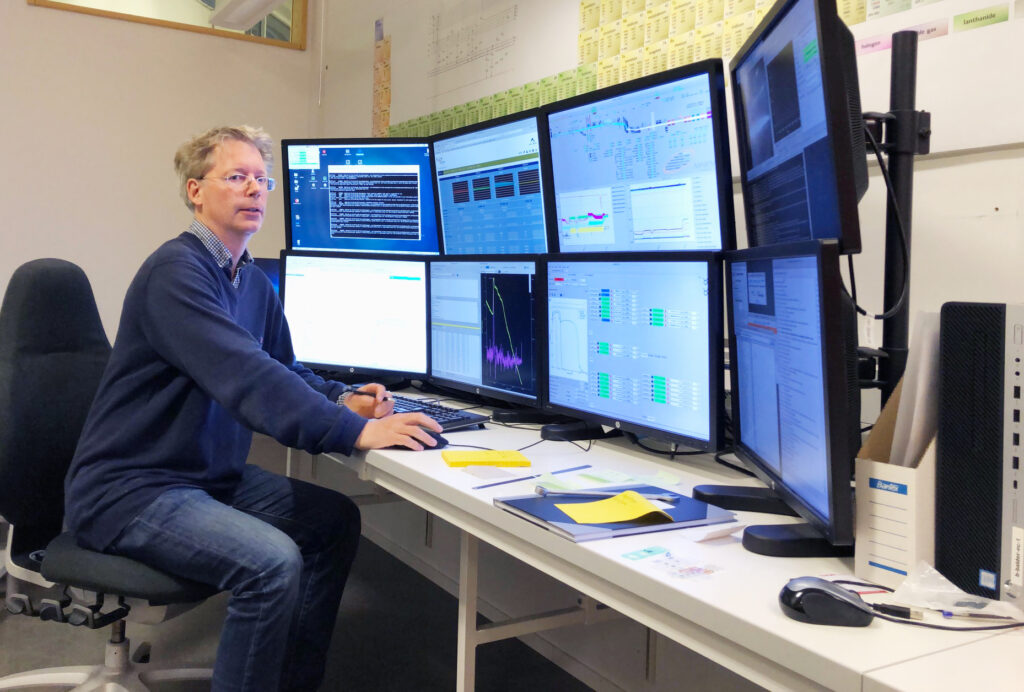
“One of the most interesting MAX-phase and MXene system is Ti3AlC2 and the Ti3C2Tx,” continues Magnuson. Lars-Åke Näslund, researcher at Linköping University adds “In the MAX-phase, titanium and carbon atoms form three monolayers of titanium and two monolayers of carbon in an alternated sequence resulting in 2D Ti3C2-layers, which are alternated with monolayers of Al.”
The MXene sheets are functionalised, referring to the outermost Tx atoms giving the material-specific function. The Tx of the Ti3C2Tx MXene in the study is a combination of fluoride and oxygen.
“The key for functionalisation of the surfaces for specific applications is the understanding of the termination species and their bonding nature toward the MXene you’re trying to make,” explains Magnuson. “There are more than 150 MAX-phases and more than 30 MXenes, which offer properties that may be useful in many different applications such as composite materials with high strength, fuel- and solar cells, 2D-based electronics, and energy storage systems.”
State-of-the-art beamline
The researchers used beamline Balder to investigate how the atoms in the MXene and MAX-phase materials are bound to each other.
“The physical properties of the MAX-phases and the MXenes depend on the chemical bonding environment, but more importantly between the Ti3C2-layers and the Al monolayers in the MAX-phase and between the Ti3C2-layers and the termination species in the MXene, respectively,” says Magnuson. “The aim of the present study was to improve the understanding of the nature of the surface termination in terms of coordination and bond lengths as well as the electronic structure and chemical state properties of Ti for Ti3AlC2 and Ti3C2Tx.”
They used X-ray Absorption Near-Edge Spectroscopy (XANES) and Extended X-ray Absorption Fine Structure (EXAFS) spectroscopy.
“XANES probes the unoccupied density of states of the absorbing atoms and is, therefore, an ideal technique for determining the chemical surroundings and local bonding structure around the transition metal. In addition, EXAFS provides information about the chemical coordination and atom distances around the probed element.”, says Magnuson. “The work we have done also shows that the state-of-the-art Balder beamline provides highly relevant contributions in the field of application-inspired 2D materials.”
Investigations of bonding
“The XANES results suggest slightly weaker Ti-C bonds in Ti3C2Tx compared to Ti3AlC2,” adds Näslund. “We could also resolve the crystal-field splitting in titanium of our samples with XANES. Nobody has seen this before.”
Crystal-field splitting is a tiny splitting of the electronic energy levels that happens when transition metal atoms are arranged in a crystal. The researchers also wanted to investigate the termination, Tx, atoms after heating the material.
“We have investigated the MAX phase material Ti3AlC2 and the MXene material Ti3C2Tx, where the latter was examined before and after a series of heat treatments,” says Magnuson. “The Ti-C bond strengths in the Ti3C2-layers are altered when the stronger interacting F and O in Ti3C2Tx replace the Al-monolayer in Ti3AlC2. In addition, we also found significant changes in the Ti-O/F coordination on the Ti3C2Tx surfaces before and after the heat treatment to 750 °C.”
Exciting plans ahead
“It’s exciting that we could perform the temperature-dependent in-situ XANES and EXAFS measurements in a gas cell at the Balder beamline,” concludes Magnuson. “There is still much to learn about the chemical bonding environment in Ti3AlC2 and Ti3C2Tx. In particular, the knowledge about the charge-transfer, bonding interaction, and bond lengths between the Ti3C2-layers and the termination species is very limited. Now, we plan to study other MXenes and 2D materials with different elements at Balder and use other complementary techniques such as resonant- and angular-resolved photoemission spectroscopy at MAX IV.”